HIF Pathway and Cancer
Abstract
HIF-1 is a transcription factor that plays a critical role in the response to hypoxia and is overexpressed in many types of cancer. It regulates a wide range of genes involved in tumor angiogenesis, metabolism, and metastasis, making it an attractive target for cancer therapy. Clinical trials targeting HIF-1 inhibitors have shown promising results, although challenges and limitations in the development of HIF-1 inhibitors exist, such as the lack of selectivity and potency of current inhibitors, and the need for predictive biomarkers to identify patients who will benefit from treatment. In addition, the development of combination therapies and imaging techniques to monitor HIF-1 expression and activity in real time is also needed.
Introduction of Transcription Factors and HIF-1
Transcription factors play a crucial role in cancer development and progression. These are proteins that bind to specific DNA sequences and regulate gene expression by turning genes on or off. By controlling the expression of genes involved in key cellular processes such as cell division, cell differentiation, and apoptosis, transcription factors can influence tumor initiation, growth, and metastasis. One such transcription factor that has been extensively studied in the context of cancer is hypoxia-inducible factor-1 (HIF-1)[1]. HIF-1 is a heterodimeric protein complex composed of two subunits: HIF-1α and HIF-1β. HIF-1α is regulated by oxygen levels and is stabilized under hypoxic conditions, whereas HIF-1β is constitutively expressed. Upon stabilization, HIF-1α translocates to the nucleus, where it forms a complex with HIF-1β and binds to hypoxia response elements (HREs) in the promoters of target genes, leading to their upregulation. HIF-1 is a key regulator of the cellular response to hypoxia and has been shown to be upregulated in a variety of solid tumors, including breast, lung, prostate, and colon cancer[2]. In cancer cells, HIF-1 promotes angiogenesis, metabolic reprogramming, and invasion and metastasis, all of which are critical for tumor growth and progression. One of the major functions of HIF-1 in cancer is its role in tumor angiogenesis. Under hypoxic conditions, HIF-1 induces the expression of vascular endothelial growth factor (VEGF) and other pro-angiogenic factors, which promote the formation of new blood vessels that supply nutrients and oxygen to the growing tumor. In addition, HIF-1 also promotes the expression of matrix metalloproteinases (MMPs), which degrade the extracellular matrix and facilitate tumor invasion and metastasis.[3] HIF-1 has a crucial role in driving metabolic reprogramming in cancer cells. In hypoxic conditions, HIF-1 activates genes responsible for glucose uptake and glycolysis, which boosts the production of ATP and cell survival. This metabolic shift towards glycolysis, despite the presence of oxygen, is a characteristic feature of many cancers, known as the Warburg effect. Apart from this, HIF-1 also enhances the expression of genes responsible for other metabolic pathways, including the pentose phosphate pathway and the tricarboxylic acid (TCA) cycle, which play significant roles in biomass synthesis and redox regulation. HIF-1 has a significant role in invasion and metastasis in cancer[4]. It induces the expression of genes related to epithelial-to-mesenchymal transition (EMT), which enables cancer cells to separate from the primary tumor and invade neighboring tissues. HIF-1 also activates genes responsible for cell migration and adhesion, along with the expression of MMPs that aid in tumor invasion and metastasis. Given its critical role in cancer development and progression, HIF-1 has emerged as a promising target for cancer therapy. Various approaches have been used to target HIF-1, including small-molecule inhibitors and RNA interference-based strategies. However, targeting HIF-1 for cancer therapy is challenging, as it is involved in multiple cellular processes and has a complex regulatory network. In addition, targeting HIF-1 may have off-target effects on normal tissues, and may be subject to resistance mechanisms.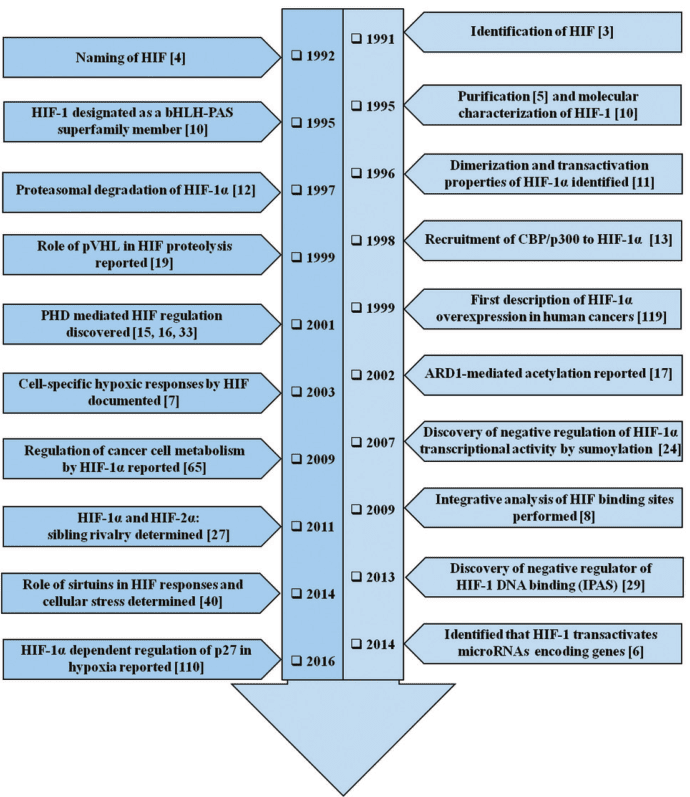
HIF-1 structure and function
Hypoxia-inducible factor-1 (HIF-1) is a transcription factor that is made up of two subunits, HIF-1α and HIF-1β, which form a heterodimeric complex. HIF-1α functions as a regulatory subunit, containing two transactivation domains (TADs) that are responsible for the recruitment of coactivator proteins and a basic helix-loop-helix (bHLH) domain that facilitates DNA binding. On the other hand, HIF-1β serves as a constitutive subunit and has a nuclear localization signal (NLS) and a PAS (Per-Arnt-Sim) domain that is crucial for dimerization with HIF-1α. Once HIF-1α and HIF-1β form a dimer, they create an active transcription factor complex that binds to hypoxia response elements (HREs) situated in the promoters of target genes.Figure 2 Structure and fate of the hypoxic master regulator[5]
The activity of HIF-1 is regulated by a variety of mechanisms, including oxygen-dependent and oxygen-independent pathways. Under normal oxygen conditions, HIF-1α is hydroxylated at specific proline residues by prolyl hydroxylase domain (PHD) proteins, which use oxygen as a substrate. Hydroxylated HIF-1α is recognized by the von Hippel-Lindau (VHL) tumor suppressor protein, which targets HIF-1α for ubiquitination and proteasomal degradation. In this way, HIF-1α levels are kept low under normoxic conditions. In contrast, under hypoxic conditions, PHD activity is inhibited, leading to the stabilization of HIF-1α. Stabilized HIF-1α translocates to the nucleus, where it forms a complex with HIF-1β and binds to HREs in the promoters of target genes. The binding of HIF-1 to HREs results in the upregulation of genes involved in key cellular processes such as angiogenesis, metabolism, and cell survival. Apart from the oxygen-dependent pathway, HIF-1 activity can be controlled by pathways that are not oxygen-dependent. The phosphorylation and stabilization of HIF-1α can be induced by growth factor signaling pathways, such as the PI3K/Akt/mTOR pathway[6,7], which leads to the activation of HIF-1. Epigenetic mechanisms such as histone acetylation and methylation can also regulate HIF-1 by modifying the accessibility of HIF-1 binding sites in the promoters of target genes. HIF-1 plays a crucial role in oxygen sensing and response, and one of its primary functions is to induce the expression of genes involved in angiogenesis and glycolysis under hypoxic conditions. In particular, HIF-1 promotes the expression of genes such as vascular endothelial growth factor (VEGF) and platelet-derived growth factor (PDGF) that are critical for angiogenesis, facilitating the formation of new blood vessels to supply oxygen and nutrients to hypoxic tissues. Furthermore, HIF-1 also promotes the expression of genes such as glucose transporters (GLUTs) and glycolytic enzymes, which facilitate glycolysis and enable cells to produce ATP even in the absence of oxygen.[8] HIF-1 plays a significant role in the regulation of cellular metabolism, beyond promoting glycolysis. It can also promote the expression of genes involved in other metabolic pathways, such as the pentose phosphate pathway and the TCA cycle, which are vital for biomass synthesis and redox regulation. The regulation of cellular metabolism by HIF-1 has implications for cell growth, survival, and differentiation. Furthermore, HIF-1 is also involved in the regulation of cell survival and apoptosis. In hypoxic conditions, HIF-1 promotes cell survival by upregulating the expression of anti-apoptotic genes such as Bcl-2 and Bcl-xL, and by reducing the expression of pro-apoptotic genes such as Bax and Bad. As a result, HIF-1 helps to protect cells from cell death caused by hypoxia.HIF’s roles in cancer
Hypoxia-inducible factor-1 (HIF-1) plays a critical role in cancer, as it promotes tumor progression and metastasis by regulating a wide range of genes involved in angiogenesis, metabolism, and cell survival. The expression of HIF-1 is upregulated in many types of cancer, and its expression levels have been correlated with poor prognosis and reduced survival in patients. One of the primary ways in which HIF-1 promotes tumor progression is through its role in tumor angiogenesis. HIF-1 promotes angiogenesis by upregulating the expression of pro-angiogenic genes such as vascular endothelial growth factor (VEGF) and platelet-derived growth factor (PDGF), which promote the formation of new blood vessels to supply oxygen and nutrients to hypoxic tumors. By promoting angiogenesis, HIF-1 helps tumors to grow and metastasize, and it can also contribute to the development of resistance to anti-cancer therapies. HIF-1 has a crucial role not only in angiogenesis but also in the metabolic regulation of cancer. In the absence of oxygen, HIF-1 facilitates the expression of genes associated with glycolysis, such as glucose transporters (GLUTs) and glycolytic enzymes, to provide ATP for cancer cells. This shift in cellular metabolism, termed the Warburg effect, is a characteristic feature of cancer and is responsible for the survival and growth of cancer cells in hypoxic conditions. Furthermore, HIF-1 can also regulate other metabolic pathways such as the pentose phosphate pathway and the TCA cycle, which are critical for biomass synthesis and redox regulation. Furthermore, HIF-1 plays a crucial role in the metastasis of cancer, whereby cancer cells spread from the primary tumor to other parts of the body. HIF-1 can enhance metastasis by promoting the expression of genes responsible for epithelial-mesenchymal transition (EMT), a process that confers greater mobility and invasiveness to cancer cells. HIF-1 can also increase the expression of genes involved in the remodeling of the extracellular matrix (ECM), including matrix metalloproteinases (MMPs), which facilitate the penetration and invasion of cancer cells into neighboring tissues. The involvement of HIF-1 in cancer is intricate and diverse, as it can facilitate tumor growth and metastasis through various mechanisms. HIF-1 expression is increased in various cancer types, such as breast, lung, prostate, and pancreatic cancer, among others. In certain cases, HIF-1 overexpression has been linked to unfavorable prognosis and reduced patient survival rates. Since HIF-1 plays a crucial role in cancer, it is an appealing target for anti-cancer therapies. Multiple strategies have been proposed to target HIF-1 in cancer, including small molecule inhibitors of HIF-1 activity and HIF-1-targeted gene therapy. However, developing effective HIF-1 inhibitors has been challenging, as HIF-1 regulates numerous cellular processes that are crucial for normal physiology and disease.Clinical trials targeting HIF-1
Hypoxia-inducible factor-1 (HIF-1) is an attractive target for cancer therapy, given its critical role in tumor progression and metastasis. Over the past few decades, several HIF-1 inhibitors have been developed and tested in preclinical studies, with promising results. However, the translation of HIF-1 inhibitors to the clinic has been challenging, with several limitations and obstacles that need to be overcome.Table 1 Major chemical inhibitors of HIF-1 activity[5]
Summary and future directions
HIF-1 is a transcription factor that plays a critical role in the response to hypoxia and is overexpressed in many types of cancer. It regulates a wide range of genes involved in tumor angiogenesis, metabolism, and metastasis, making it an attractive target for cancer therapy. Clinical trials targeting HIF-1 inhibitors have shown promising results, although challenges and limitations in the development of HIF-1 inhibitors exist, such as the lack of selectivity and potency of current inhibitors, and the need for predictive biomarkers to identify patients who will benefit from treatment. In addition, the development of combination therapies and imaging techniques to monitor HIF-1 expression and activity in real time is also needed. Future research in the field of HIF-1 and cancer therapy could address several key challenges. For instance, there is a need to develop more selective and potent HIF-1 inhibitors that do not affect other transcription factors or members of the HIF family. Moreover, predictive biomarkers could be identified to guide patient selection, and combination therapies may be investigated to enhance the efficacy of HIF-1 inhibitors. Further research could also explore the mechanisms of HIF-1 activation and regulation, as well as the crosstalk between HIF-1 and other signaling pathways in cancer, to develop novel therapeutic strategies. In addition, non-invasive imaging techniques that can monitor HIF-1 expression and activity in real-time could be developed to guide personalized cancer therapy. Precision medicine approaches, such as genomics and proteomics, could also be employed to identify patient-specific alterations in HIF-1 signaling pathways, leading to more personalized cancer therapy. Overall, future research in HIF-1 and cancer therapy aims to develop more effective and personalized treatments that target the complex signaling networks involved in cancer development and progression, with the potential to improve patient outcomes.Reference
- Semenza, G. L. (2014). Oxygen sensing, hypoxia-inducible factors, and disease pathophysiology. Annual Review of Pathology: Mechanisms of Disease, 9, 47-71.
- Camps, C., Saini, H. K., Mole, D. R., Choudhry, H., Reczko, M., Guerra-Assunção, J. A., … & Ragoussis, J. (2014). Integrated analysis of microRNA and mRNA expression and association with HIF binding reveals the complexity of microRNA expression regulation under hypoxia. Molecular cancer, 13(1), 1-21.
- Kelly, B. D., Hackett, S. F., Hirota, K., Oshima, Y., Cai, Z., Berg-Dixon, S., … & Semenza, G. L. (2003). Cell type–specific regulation of angiogenic growth factor gene expression and induction of angiogenesis in nonischemic tissue by a constitutively active form of hypoxia-inducible factor 1. Circulation research, 93(11), 1074-1081.
- Balamurugan, K. (2016). HIF‐1 at the crossroads of hypoxia, inflammation, and cancer. International journal of cancer, 138(5), 1058-1066.
- Soni, S., & Padwad, Y. S. (2017). HIF-1 in cancer therapy: two decade long story of a transcription factor. Acta oncologica, 56(4), 503-515.
- Sun, Q., Chen, X., Ma, J., Peng, H., Wang, F., Zha, X., … & Zhang, H. (2011). Mammalian target of rapamycin up-regulation of pyruvate kinase isoenzyme type M2 is critical for aerobic glycolysis and tumor growth. Proceedings of the National Academy of Sciences, 108(10), 4129-4134.
- Agani, F., & Jiang, B. H. (2013). Oxygen-independent regulation of HIF-1: novel involvement of PI3K/AKT/mTOR pathway in cancer. Current cancer drug targets, 13(3), 245-251.
- Azoitei, N., Becher, A., Steinestel, K., Rouhi, A., Diepold, K., Genze, F., … & Seufferlein, T. (2016). PKM2 promotes tumor angiogenesis by regulating HIF-1α through NF-κB activation. Molecular cancer, 15, 1-15.