Optimizing Cancer Treatment: Overcoming Challenges in Combination Chemotherapy and Cell Cycle Regulation
Abstract
Combination therapies that target both chemotherapy and cell cycle regulation have emerged as a promising strategy to enhance cancer treatment efficacy. By synchronizing tumor cells and modulating cell cycle checkpoints, these approaches can increase the susceptibility of cancer cells to chemotherapeutic drugs, improve drug delivery, and overcome resistance mechanisms. However, challenges such as tumor heterogeneity, drug resistance, and the precise timing of drug administration continue to limit the full potential of combination therapies. Personalized medicine and targeted drug delivery systems offer future directions to improve therapeutic outcomes by addressing these challenges. This review highlights the current obstacles in combination chemotherapy and cell cycle regulation and discusses promising strategies for overcoming them.
Introduction to Cell Cycle Regulation and Cancer Therapy
The cell cycle is a tightly regulated process that governs cell division and proliferation. It involves a series of stages—G1, S, G2, and M phases—each of which is controlled by a complex network of proteins, enzymes, and signaling pathways. These regulatory mechanisms ensure that cells divide only when necessary and that any errors in DNA replication or division are corrected. Disruptions in this regulation, however, can lead to uncontrolled cell proliferation, a hallmark of cancer. Tumor cells often exhibit abnormal cell cycle regulation, allowing them to bypass growth control mechanisms and persist despite cellular damage. Cell cycle regulation plays a crucial role in the sensitivity of tumor cells to chemotherapy. Chemotherapeutic drugs are designed to target rapidly dividing cells, making cancer cells more vulnerable. These drugs can act on various stages of the cell cycle, interfering with DNA replication, mitosis, or cell division. For example, some chemotherapeutic agents are phase-specific, meaning they are most effective at particular points in the cell cycle. Others, known as cell cycle-nonspecific agents, can act throughout the entire cycle. Despite their efficacy, chemotherapy often fails to eliminate all cancer cells, particularly those in resting phases or those that have developed resistance mechanisms. otherapy is currently one of the main methods of tumor treatment . However, there are still several obstacles to achieve the desired therapeutic effect. Some inevitable side effects such as nausea and hair loss usually accompany chemotherapy. In the course of chemotherapy, the multidrug resistance (MDR) of tumor cells will also affect the treatment. As a single chemotherapeutic drug usually cannot meet the requirements of clinical treatment, the combination of different chemotherapeutic drugs or treatments (e.g., chemotherapy and radiotherapy) is operated to prevent the replication, invasion, and metastasis of cancer cells. These strategies are of great significance for reducing side effects, overcoming MDR, reducing the dosage of each drug, and finally improving the therapeutic effect. In conclusion, the intricate relationship between cell cycle regulation and chemotherapy underscores the potential for combined treatment strategies. As research advances, optimizing these interactions could lead to more targeted and effective cancer therapies.Mechanisms of Chemotherapeutic Drugs
Chemotherapeutic drugs work by interfering with the cell cycle and targeting rapidly dividing cells, a key characteristic of cancer. These drugs can be classified into two main categories based on their action: cell cycle-specific and cell cycle-nonspecific agents. Understanding the mechanisms of these drugs is crucial for optimizing their effectiveness and minimizing resistance. Cell cycle-specific drugs are most effective at particular stages of the cell cycle. For instance, antimetabolites like methotrexate and 5-fluorouracil (5-FU) act during the S-phase, inhibiting DNA synthesis and replication. These drugs mimic natural nucleotides, incorporating themselves into DNA during replication and causing cell death. Similarly, topoisomerase inhibitors, such as irinotecan and etoposide, interfere with the action of topoisomerases, enzymes that are essential for DNA unwinding during replication. These inhibitors are active during the S and G2 phases of the cell cycle, causing DNA breaks that prevent the proper replication of cancer cells. On the other hand, cell cycle-nonspecific drugs can affect cells at any stage of the cell cycle. Drugs such as alkylating agents (e.g., cyclophosphamide and cisplatin) work by adding alkyl groups to the DNA, leading to crosslinking and strand breakage, which ultimately disrupts DNA replication and transcription. These agents are particularly useful in treating solid tumors, as they can target cells in both dividing and resting phases of the cell cycle. Despite their potency, chemotherapy drugs often have limitations due to tumor cell heterogeneity and resistance mechanisms. For example, cells in the G0 phase (resting phase) are less susceptible to cell cycle-specific drugs, which only target proliferating cells. Additionally, cancer cells can develop resistance to chemotherapy through various mechanisms, including drug efflux, DNA repair activation, or altered drug metabolism, which reduces the overall therapeutic efficacy. In conclusion, understanding the mechanisms of chemotherapeutic drugs is essential for improving treatment strategies. Combining cell cycle-specific drugs with other therapies or targeting resistance mechanisms may offer promising solutions to enhance chemotherapy effectiveness.The Link Between Cell Cycle Regulation and Tumor Development
DNA damage response (DDR) mechanisms play a critical role in regulating the cell cycle after DNA damage, often through cell cycle checkpoints, which halt progression and allow for DNA repair. ATR (ataxia-telangiectasia and Rad3-related protein) and PARP (poly(ADP-ribose) polymerase) are key DNA repair proteins that activate checkpoints in the S-phase and G2/M phases under replication stress (RS), blocking cell cycle progression to facilitate repair. Inhibitors of ATR and PARP interfere with these DNA repair processes, enhancing the efficacy of chemotherapy treatments. These inhibitors have been increasingly studied for their potential to improve therapeutic outcomes when used in combination with DNA-damaging chemotherapeutic agents, such as alkylating agents. Several targeted cell cycle inhibitors have been identified as promising agents for enhancing cancer treatment. Withaferin A (WA), for instance, inhibits ATR and its downstream kinase, CHK1, leading to G2/M phase arrest in tumor cells. When combined with platinum-based drugs, WA has been shown to reduce both chemotherapy resistance and toxicity, demonstrating strong efficacy in 3D-cultured breast cancer cells. nLs-BG129, a nano-liposome ATR inhibitor, is another promising compound that enhances the antitumor activity of chemotherapeutic agents like gemcitabine (GEM) and carboplatin, by improving stability and extending clearance time in vivo. Diallyl disulfide (DADS), a natural organic sulfide, acts as a DNA repair inhibitor by targeting the proteins involved in DNA resection, such as Sae2 and Exo1. DADS has been shown to stimulate cell cycle arrest, promote apoptosis, and prevent tumor invasion and angiogenesis. Other ATR inhibitors, including M6620, M4344, AZD6738, and BAY1895344, are currently in clinical development, demonstrating significant potential when combined with chemotherapeutic agents like platinum drugs (e.g., cisplatin, carboplatin) and topoisomerase inhibitors. The combination of ATR inhibitors with platinum-based drugs has shown promising results in clinical trials. For example, the ATR inhibitor VE-822 combined with oxaliplatin has demonstrated strong synergy across multiple colorectal cancer cell lines, including oxaliplatin-resistant subclones. This combination promotes the formation of DNA strand breaks (SSBs and DSBs), induces cell cycle arrest, and triggers apoptosis. Moreover, combining VE-822 with topoisomerase I inhibitors like irinotecan has enhanced the sensitivity of triple-negative breast cancer (TNBC) cells to chemotherapy by inhibiting CHK1 phosphorylation and overcoming chemotherapy resistance. In addition to ATR inhibitors, PARP inhibitors are another class of drugs showing significant promise when combined with chemotherapy. Olaparib, niraparib, and velaparib are well-known PARP inhibitors, and their combination with alkylating agents has led to synergistic cytotoxic effects in cancer treatments. Inhibition of PARP prevents the recruitment of repair enzymes to damaged replication forks, effectively trapping PARP-1 at the site of DNA damage and further hindering DNA repair processes.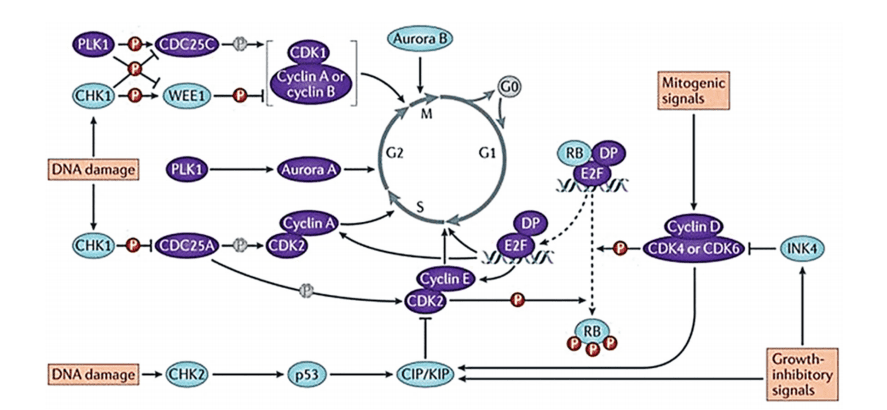
Figure 1. Evolution of the cell cycle and the main regulator proteins.
These combination therapies have been extensively studied in various cancers, including ovarian, breast, small cell lung carcinoma (SCLC), and pancreatic cancer, showing increased sensitivity to chemotherapeutic drugs and reduced resistance. The use of checkpoint inhibitors, including Prexasertib (LY2606368), AZD7762, and others, further supports the approach of inhibiting cell cycle checkpoints to enhance the effectiveness of DNA-damaging chemotherapeutic agents, ultimately leading to improved tumor suppression and treatment efficacy.Combination Strategies: Chemotherapy and Cell Cycle Regulation
Combination strategies that target both chemotherapy and cell cycle regulation have emerged as promising approaches to improve cancer treatment efficacy. While traditional chemotherapy primarily targets rapidly dividing cells, integrating cell cycle regulation into treatment can enhance drug sensitivity, overcome resistance, and address the heterogeneous nature of tumors. By modulating key cell cycle checkpoints, researchers aim to make cancer cells more susceptible to chemotherapy or to exploit vulnerabilities in tumor cell proliferation. One strategy involves cell cycle synchronization, where cells are induced to synchronize at specific phases of the cycle, typically the G1 or G2/M phase. Chemotherapy drugs that are effective at certain stages can then be administered during these synchronized windows to maximize their effectiveness. For example, drugs that target the S-phase, like antimetabolites, can be used in combination with agents that block cells in the G1 phase, improving drug uptake and DNA replication inhibition. Another approach is to target cell cycle checkpoints directly. Tumor cells often exhibit defective checkpoints, allowing them to bypass normal cell cycle control. Inhibiting checkpoint kinases such as CHK1 or CHK2, which are responsible for halting the cycle in response to DNA damage, can force cancer cells into mitosis with unrepaired DNA, leading to cell death. This strategy can sensitize tumor cells to DNA-damaging chemotherapeutic agents such as platinum-based drugs, which would otherwise be ineffective against checkpoint-proficient cells. Targeting CDKs is also a promising strategy. Cyclin-dependent kinase inhibitors, such as palbociclib, have shown potential in slowing down the progression of certain cancers by blocking the G1/S transition. When combined with chemotherapy, these inhibitors can enhance the accumulation of cells in the G1 phase, thereby increasing their susceptibility to DNA-damaging agents. Despite the promise of these combination strategies, challenges remain, including the development of resistance mechanisms and the need for precise timing of drug administration. However, ongoing research into optimizing cell cycle regulation alongside chemotherapy offers hope for more effective, personalized cancer therapies.Challenges and Future Directions in Combination Cancer Therapy
Most chemotherapeutics act on cells in the proliferative phase. Therefore, reasonable artificial regulation of the cell cycle will have a positive impact on chemotherapy. Clinically, for solid tumors, surgery or radiotherapy is usually used to reduce the tumor volume first, and then remove the remaining cancer cells through chemotherapy. Surgery or radiotherapy will stimulate dormant tumor cells to re-enter the replication stage, and the cell cycle-specific chemotherapy drugs that follow will wipe them out. It is meaningful to further develop new coordination strategies while simplifying the treatment steps while improving the effect. Drug resistance is another significant challenge in combination cancer therapy. Over time, cancer cells can develop resistance mechanisms, such as enhanced DNA repair, drug efflux, or alterations in drug targets. For example, cancer cells may activate alternative cell cycle checkpoints to bypass the effects of chemotherapy, or they may upregulate efflux pumps that expel chemotherapeutic agents before they can exert their effect. Combining chemotherapy with agents that target these resistance mechanisms, such as checkpoint inhibitors or drug transporter inhibitors, could improve treatment outcomes. However, the development of such dual-target strategies remains an area of active research. Furthermore, the timing and sequencing of combination therapies pose additional complexities. Administering chemotherapy and cell cycle regulators in the correct order and at the right doses is critical for maximizing their synergistic effects. Too much emphasis on synchronizing cell cycles may inadvertently cause toxicity to normal cells, while incorrect timing of drug administration can diminish the potential benefits. Therefore, precise scheduling and patient monitoring are essential to avoid adverse outcomes. Looking forward, advances in targeted drug delivery systems and biomarker-driven therapies offer new avenues for improving the effectiveness of combination therapies. By using nanoparticles or other targeted delivery methods, drugs can be directed more specifically to tumor cells, reducing toxicity and increasing therapeutic efficacy. Additionally, the identification of biomarkers for specific cell cycle alterations in tumors may allow for more precise targeting of therapies, leading to better patient outcomes with fewer side effects.References
- Chien, H. W., & Chou, C. Y. (2020). Cell cycle regulation in cancer. Frontiers in Molecular Biosciences, 7, 174.
- O’Connor, M. J. (2015). Targeting the DNA damage response in cancer. Molecular Cell, 60(4), 547-560.
- Santarius, T., Shipman, S. L., & Choudhury, A. (2018). The role of cell cycle regulation in cancer therapy. Nature Reviews Cancer, 18, 361-379.
- Goulart, E., & García, G. (2019). Chemotherapy and its effects on the cell cycle. Cancer Treatment Reviews, 75, 36-42.
- Zhang, H., & Fu, S. (2019). Targeting the cell cycle to improve chemotherapy efficacy. Cancers, 11(5), 789.
- Yan, J., & Zhang, X. (2020). Chemotherapy and cell cycle regulation in cancer treatment: An overview. Journal of Clinical Medicine, 9(2), 432.
- Li, Q., & Wang, L. (2021). Exploring the synergistic effects of chemotherapy and cell cycle regulation in cancer therapy. Cancer Biology & Therapy, 22(1), 5-12.
- Kummar, S., & Chen, M. (2020). Targeting the cell cycle in cancer therapy: Opportunities and challenges. Cancer Research, 80(7), 1303-1312.
- Zhang, W., & Li, Y. (2021). Cell cycle synchronization and chemotherapy: A combined approach to improving cancer treatment. Journal of Clinical Oncology, 39(15), 1834-1843.
- Lee, M., & Lee, J. (2019). Overcoming cancer drug resistance: The role of cell cycle regulation. Cell Cycle, 18(5), 538-549.
- Zhang, J., & Zhao, S. (2022). Chemotherapy resistance and the cell cycle: Targeting key regulatory pathways. Journal of Cancer Research and Clinical Oncology, 148(7), 1489-1503.
- Zhang, H., & Wang, Y. (2019). Mechanisms of chemotherapy resistance in cancer: Advances and challenges. Cancer Treatment Reviews, 75, 12-18.
- Liu, Y., & Yang, J. (2020). Cell cycle and chemotherapy: Understanding the role of cell cycle regulation in anticancer drug development. Journal of Cancer Research, 21(5), 467-474.
- Li, Q., & Li, X. (2021). The role of chemotherapeutic agents in cell cycle arrest and apoptosis in cancer therapy. Current Cancer Drug Targets, 21(6), 410-421.
- Malik, S. A., & O’Connor, M. J. (2020). Cell cycle regulation and its impact on cancer development. Journal of Clinical Oncology, 38(12), 1568-1579.
- Wang, L., & Xu, Z. (2018). Disruption of cell cycle regulation and its role in cancer progression. Cancer Research, 78(6), 1209-1217.
- Fu, J., & Liu, W. (2019). The cell cycle and its regulation in cancer. Cancer Cell, 35(3), 359-375.
- Zhang, L., & Yu, Z. (2021). Overcoming drug resistance in cancer therapy: Targeting cell cycle checkpoints and DNA repair pathways. Frontiers in Pharmacology, 12, 755.
- Smith, M. A., & Wilson, K. (2020). Challenges in combination chemotherapy: Tumor heterogeneity and resistance mechanisms. Cancer Drug Resistance, 3(1), 45-58.
- Lee, J., & Park, S. (2022). Optimizing combination therapies in cancer treatment: Focus on cell cycle regulators and chemotherapy. Journal of Cancer Research and Clinical Oncology, 148(5), 1127-1139.