Overcoming Drug Resistance in Nucleoside-Based Anticancer Therapies: Strategies and Future Directions
Abstract
Nucleoside-based anticancer drugs have been central to cancer treatment for several decades, acting primarily by disrupting DNA and RNA synthesis in rapidly dividing cancer cells. Despite their effectiveness, a significant challenge in their clinical application is the development of drug resistance. Mechanisms such as altered drug transport, enhanced drug efflux, and the upregulation of DNA repair pathways allow cancer cells to evade the effects of these therapies, reducing their long-term efficacy. To address these challenges, researchers have explored various strategies, including combining nucleoside analogs with other agents, developing novel analogs that bypass resistance mechanisms, and utilizing targeted therapies. The future of these treatments lies in personalized medicine, with advancements in biomarker discovery and combination therapies poised to significantly improve outcomes in resistant cancers. New drug formulations and the incorporation of immunotherapies are expected to offer promising solutions to enhance the effectiveness of nucleoside-based treatments.
Introduction to Nucleoside-based Anticancer Drugs
Nucleoside-based anticancer drugs, often referred to as nucleoside analogs, have been fundamental in the treatment of cancer since their introduction in the mid-20th century. These drugs mimic the natural building blocks of DNA and RNA, effectively interrupting essential cellular processes like DNA synthesis and repair, which are critical for cancer cell survival and proliferation. As potent antimetabolites, they integrate into the cellular machinery, causing errors in DNA replication and leading to cancer cell death. Nucleoside analogs are used to treat a variety of cancers, including hematological malignancies such as leukemia, as well as solid tumors like pancreatic and breast cancer. The first successful use of a nucleoside-based drug in cancer treatment was in 1950 with the synthesis of 6-mercaptopurine, which was primarily used to treat acute leukemia. Over the decades, numerous other nucleoside analogs have been developed, including 5-fluorouracil and gemcitabine, which have shown remarkable efficacy against solid tumors. These drugs work by inhibiting enzymes involved in nucleotide synthesis or by being incorporated into the DNA of rapidly dividing cells, thereby halting cell division. Despite their effectiveness, the clinical utility of nucleoside analogs is often limited by the development of drug resistance. Cancer cells can adapt to the presence of these drugs by altering drug uptake, enhancing drug elimination, or repairing the DNA damage caused by the drugs. This makes the treatment less effective over time, prompting ongoing research into novel therapeutic strategies to overcome resistance. Nucleoside-based drugs continue to be a cornerstone in chemotherapy regimens, offering hope to patients battling various types of cancer. Their ongoing development and combination with newer treatment strategies, such as immunotherapy, remain a promising area of research in cancer treatment.Mechanisms of Action of Nucleoside-based Anticancer Drugs
Nucleoside-based anticancer drugs function by disrupting critical cellular processes required for DNA and RNA synthesis, which ultimately leads to the death of rapidly dividing cancer cells. As analogs of natural nucleosides, these drugs mimic the structure of purines and pyrimidines, the building blocks of DNA and RNA. Once incorporated into the cellular machinery, they interfere with the normal process of nucleotide synthesis, causing errors in DNA replication or halting it altogether. This action makes them highly effective in targeting cancer cells, which rely on rapid and continuous cell division for tumor growth. One of the most widely studied mechanisms is the inhibition of key enzymes involved in nucleotide synthesis, such as ribonucleotide reductase and thymidylate synthase. Drugs like gemcitabine, a deoxycytidine analog, are phosphorylated into active triphosphates that inhibit ribonucleotide reductase, leading to a depletion of deoxyribonucleotide pools and stalling DNA synthesis. Other drugs, such as 5-fluorouracil (5-FU), inhibit thymidylate synthase, preventing the synthesis of thymidine, a nucleotide essential for DNA replication. By disrupting these pathways, nucleoside analogs prevent cancer cells from properly synthesizing DNA, which induces apoptosis or cell death. Moreover, many nucleoside analogs are incorporated into the DNA chain during replication. This incorporation creates defective DNA strands that are not functional, leading to the termination of DNA synthesis. For example, cytarabine (Ara-C), a cytosine analog, is incorporated into DNA, causing chain termination. This is particularly effective in treating leukemias, where the rapid proliferation of abnormal white blood cells is a key feature of the disease. These drugs also affect RNA synthesis by inhibiting transcription processes. For example, fludarabine disrupts RNA processing, further hampering the ability of cancer cells to survive and proliferate. By targeting these fundamental processes, nucleoside-based drugs offer a potent method of halting cancer progression, although the development of resistance remains a significant challenge in their clinical application.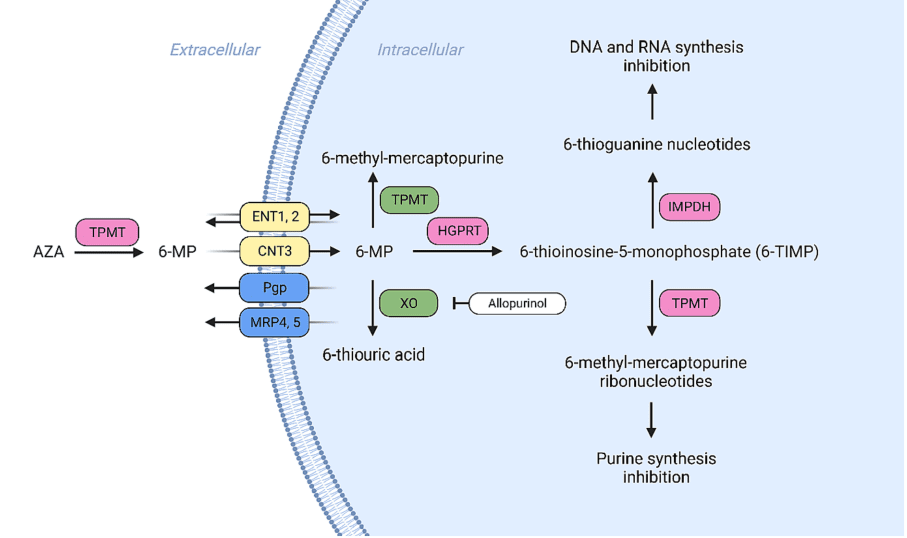
Fig. 1. Membrane transport and metabolism of 6-thioguanine (6-TG).
Strategies to Overcome Drug Resistance
Given the significant challenge posed by drug resistance in nucleoside-based cancer therapies, researchers and clinicians have developed multiple strategies to enhance the efficacy of these drugs. One of the most promising approaches is the combination of nucleoside analogs with other therapeutic agents. This strategy is aimed at overcoming specific resistance mechanisms, such as drug efflux or altered drug metabolism. For instance, 6-mercaptopurine, commonly used in leukemia treatments, can be combined with methotrexate to enhance the overall therapeutic effect and reduce the development of resistance. Similarly, gemcitabine has been effectively combined with other chemotherapeutic agents, such as cisplatin, to overcome resistance in solid tumors like pancreatic and lung cancer. Another approach involves developing novel nucleoside analogs that can bypass resistance mechanisms. Newer drugs, designed to evade cellular efflux pumps or resist deactivation by cellular enzymes, are being researched. These analogs can either be more efficient in entering the cells or be less susceptible to drug elimination processes. An example is clofarabine, a newer analog that is more resistant to enzymatic degradation and has shown effectiveness in overcoming resistance in some leukemias. Targeted therapies are also being explored. These therapies focus on inhibiting specific proteins or pathways that cancer cells rely on for survival. For instance, inhibitors targeting enzymes like ribonucleotide reductase (which gemcitabine inhibits) or molecules involved in DNA repair are being investigated. These targeted inhibitors could help enhance the sensitivity of cancer cells to nucleoside analogs, making the treatment more effective even in resistant cancers. Lastly, there is ongoing research into biomarkers that could predict which patients are more likely to develop resistance to nucleoside drugs. By identifying these patients early on, clinicians can tailor treatments more effectively and adjust therapeutic strategies to improve outcomes.The Future of Nucleoside-based Cancer Therapies
Nucleosides represent a versatile group of antimetabolites that have been successfully applied in the treatment of a broad spectrum of conditions, including diverse forms of cancer, myelodysplastic syndromes (MDSs), multiple sclerosis, and viral infections. The mechanism of action of this class of drugs typically involves the activation of a phosphorylation cascade, culminating in the formation of triphosphate forms that interact with nucleic acids. However, as these drugs take effect, cancer cells can evolve strategies to resist their impacts. While the pathways leading to resistance are diverse and multifactorial, several common pathways emerge, including a) alterations in membrane transporters, which can be characterized by reduced expression or activity of ENTs, CNTs, and/or overexpression of efflux pumps such as Pgp, MRPs, and BCRP b) reduced activity of activating kinases, which may result in decreased phosphorylation of the drug and its capacity to interact with nucleic acids; and c) accelerated drug elimination through the activation of metabolic processes or increased renal clearance (see Table 1). Alongside these mechanisms, cancer cells may also develop resistance to nucleoside drugs by activating DNA repair mechanisms. This approach enables cancer cells to repair the damage caused by nucleoside drugs, thereby reducing their effectiveness. Given these complex resistance mechanisms, several strategies for countermeasures have been proposed. These include targeted therapies, the development of innovative nucleoside drugs, and the formulation of unique drug combinations yielding synergistic effects. Such strategies hold the potential to prevent and overcome the resistant phenotype, thereby restoring tumor sensitivity and ultimately enhancing patient survival rates. The development of new strategies to combat resistance to nucleoside drugs continues to be a vibrant area of research, promising further breakthroughs in the future.References
- Hruba, L., Das, V., Hajduch, M., & Dzubak, P. (2023). Nucleoside-based anticancer drugs: Mechanism of action and drug resistance. Biochemical Pharmacology, 215, 115741.
- Marin, J. J. G., Briz, O., Rodríguez-Macias, G., Díez-Martín, J. L., Macias, R. I., Boumahdi, R., … & Ortiz-Gordillo, M. A. (2020). Molecular bases of drug resistance in hepatocellular carcinoma. Cancers (Basel), 12(6), 1–26.Clarke, S. J., Jackman, A. L., & Harrap, K. R. (1991). Antimetabolites in cancer chemotherapy. Advances in Experimental Medicine and Biology, 309, 7–13.
- Parker, W. B., & Cheng, Y. C. (1990). Metabolism and mechanism of action of 5-fluorouracil. Pharmacology & Therapeutics, 48(3), 381-395.
- Conneely, S. E., Cooper, S. L., & Rau, R. E. (2020). Use of allopurinol to mitigate 6-mercaptopurine associated gastrointestinal toxicity in acute lymphoblastic leukemia. Frontiers in Oncology, 10, 1129.
- Gesto, S., Cerqueira, M. F. S. A., Fernandes, N. A. P., & Ramos, J. M. (2012). Gemcitabine: A critical nucleoside for cancer therapy. Current Medicinal Chemistry, 19(7), 1076-1087.
- Clarke, S. J., Jackman, A. L., & Harrap, K. R. (1991). Antimetabolites in cancer chemotherapy. Advances in Experimental Medicine and Biology, 309, 7-13.
- Conroy, T., Desseigne, F., Ychou, M., Bouché, O., Guimbaud, R., Bécouarn, Y., … Ducreux, M. (2011). FOLFIRINOX versus gemcitabine for metastatic pancreatic cancer. The New England Journal of Medicine, 364(19), 1817-1825.
- Galmarini, C. M., Mackey, J. R., & Dumontet, C. (2002). Nucleoside analogues and nucleobases in cancer treatment. Lancet Oncology, 3(7), 415-424.
- Gesto, S., Cerqueira, M. F. S. A., Fernandes, N. A. P., & Ramos, J. M. (2012). Gemcitabine: A critical nucleoside for cancer therapy. Current Medicinal Chemistry, 19(7), 1076-1087.
- Parker, W. B., & Cheng, Y. C. (1990). Metabolism and mechanism of action of 5-fluorouracil. Pharmacology & Therapeutics, 48(3), 381-395.
- Plunkett, W., Huang, P., & Gandhi, V. (1995). Preclinical characteristics of gemcitabine. Anticancer Drugs, 6(Suppl 6), 7-13.
- Xu, H., Faber, C., Uchiki, T., Racca, J., & Dealwis, C. (2006). Structures of eukaryotic ribonucleotide reductase I define gemcitabine diphosphate binding and subunit assembly. Proceedings of the National Academy of Sciences, 103(11), 4028-4033.
- Yang, H., Yang, S., & Chen, X. (2020). New mechanism of gemcitabine and its phosphates: DNA polymerization disruption via 3′-5′ exonuclease inhibition. Biochemistry, 59(37), 4344-4352.