Small Molecule Prodrugs: Current Status and Future Prospects
Abstract
Prodrugs represent a significant advancement in pharmaceutical sciences by overcoming the limitations of active pharmaceutical ingredients (APIs). These chemically modified APIs enhance bioavailability, solubility, and permeability, and convert into their active forms through biotransformation. A recent review in Nature Reviews Drug Discovery analyzes small-molecule prodrugs, highlighting their development, therapeutic applications, and future potential. Prodrugs address key pharmacokinetic and pharmacodynamic challenges, improving drug profiles to offer enhanced therapeutic benefits, reduced side effects, and better patient compliance. The review showcases examples of anti-infectives, chemotherapeutics, and anti-inflammatory drugs. As the field evolves, sophisticated prodrugs aim to tackle complex drug delivery issues, paving the way for innovative treatments and personalized medicine.
Introduction to Prodrugs
Prodrugs represent a significant advancement in pharmaceutical sciences, offering a strategic approach to overcome limitations associated with active pharmaceutical ingredients (APIs). Essentially, prodrugs are chemically modified versions of APIs designed to enhance certain properties such as bioavailability, solubility, and permeability. This strategic modification allows prodrugs to undergo biotransformation within the body, converting them into their active forms. The recent review published in Nature Reviews Drug Discovery provides an in-depth analysis of the current landscape of small-molecule prodrugs, highlighting their development, therapeutic applications, and future potential. The concept of prodrugs is crucial as it addresses various pharmacokinetic and pharmacodynamic challenges that hinder the efficacy of conventional APIs. By improving the pharmacological profiles of these drugs, prodrugs can offer enhanced therapeutic benefits, reduced side effects, and improved patient compliance. This comprehensive review sheds light on the rationales behind prodrug development, showcasing examples across multiple therapeutic areas, including anti-infectives, chemotherapeutics, and anti-inflammatory drugs. As the field progresses, the development of more sophisticated prodrugs aims to tackle even more complex drug delivery issues, paving the way for innovative treatments and personalized medicine. Prodrugs are molecular derivatives of active pharmaceutical ingredients (APIs) that inherently possess minimal or no intended biological activity. However, they offer advantageous properties over their corresponding APIs, such as enhanced pharmacokinetics or diminished side effects. Following administration, prodrugs undergo biotransformation through various enzymatic and/or chemical processes to release the active APIs (Figure 1a).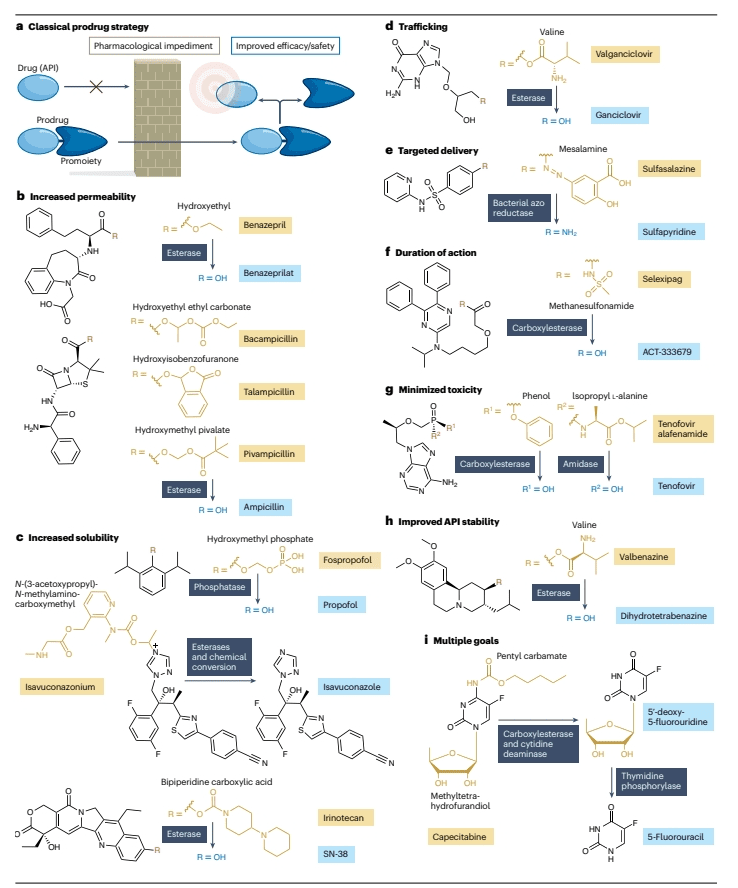
Figure 1: Structure and use of technical drug
Reasons for Prodrug Development
Improving Bioavailability: Over half of prodrugs are designed to enhance bioavailability, primarily by increasing drug penetration (35%) or solubility (15%). Targeted Drug Delivery: Approximately 24% of prodrugs aim to achieve targeted drug delivery to specific tissues, such as sulfonamides that are selectively activated by intestinal bacteria. Prolonging Duration of Action: Around 15% of prodrugs are developed to extend the duration of action of the drug, such as those that slowly hydrolyze to the active form. Minimizing Toxicity: About 7% of prodrugs are designed to reduce drug toxicity, often by ensuring the drug is primarily converted intracellularly, thereby reducing side effects. Improving API Stability: Around 6% of prodrugs are created to enhance the chemical or metabolic stability of the active pharmaceutical ingredient (API). Developing New Dosage Forms: Using the "co-drug" approach, new dosage forms are developed by combining two APIs into a single prodrug structure. Addressing Complex ADMET Challenges: Although currently approved prodrugs mainly aim to improve bioavailability, research on prodrugs increasingly focuses on addressing more complex ADMET (absorption, distribution, metabolism, excretion, and toxicity) challenges. Improving Physicochemical Properties of Drugs: By adding promoieties, the physicochemical properties of prodrugs are modified to better align with the desirable properties of successful drugs in the therapeutic area.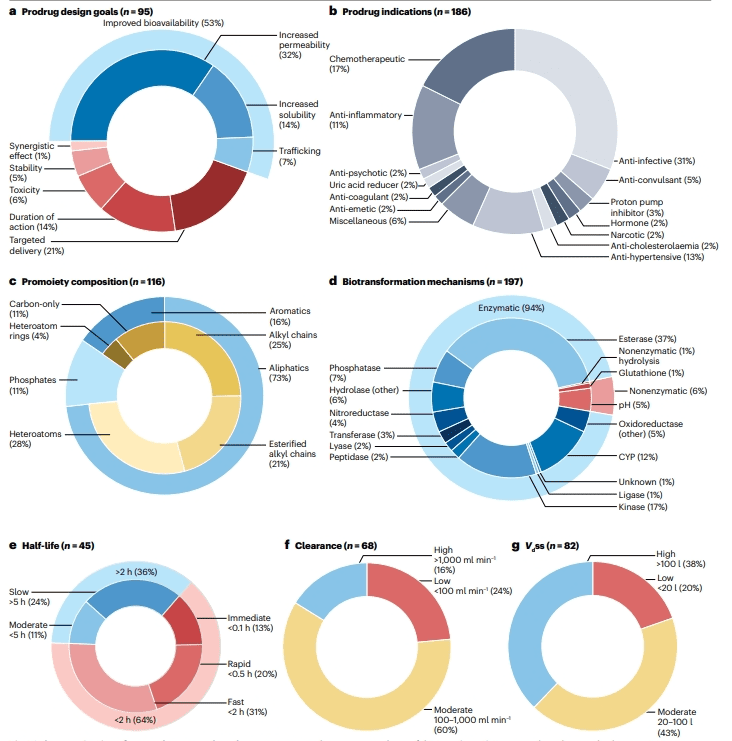
Figure 2: Characteristics of currently approved technical products
New Strategies for Prodrug Design
Addressing Antibiotic Resistance: New prodrug design strategies aim to tackle complex treatment challenges, such as antibiotic resistance. By leveraging the evolutionary adaptability of resistant microorganisms, prodrugs are developed to be effective specifically against β-lactamases, thereby minimizing harm to non-target microorganisms. Innovative Active Transport: Modern prodrug designs enhance active transport mechanisms. For instance, they increase bacterial uptake of antibiotics by incorporating side chain binding, which facilitates better delivery of the drug into bacterial cells. Targeting Disease Tissues: Prodrugs are being designed to target specific disease tissues. One approach involves using promoter groups covalently bound to serum albumin to reduce cardiotoxicity, ensuring that the drug acts primarily on the intended tissues. Brain-Targeted Prodrugs: Strategies for developing brain-targeted prodrugs include the use of redox strategies with dihydropyridine compounds. These create bio-oxidizable prodrugs that enhance the drug's ability to cross the blood-brain barrier, thereby reducing peripheral side effects. Advancements in Computational Methods: With the progress in computational methods, machine learning is poised to accelerate prodrug development. By guiding the design process, machine learning helps achieve optimal ADMET (absorption, distribution, metabolism, excretion, and toxicity) properties. Additionally, the use of metabolic models further refines the design of promoter groups to ensure appropriate drug release.Prodrug Indications
The dataset of 178 approved prodrugs revealed a total of 186 indications, as some prodrugs are approved for multiple uses. The analysis indicated that the largest category of currently approved prodrugs is anti-infectives, accounting for 33% (58 original drugs). This is followed by chemotherapeutics at 18% (32 original drugs), antihypertensives at 14% (25 original drugs), and anti-inflammatory drugs at 12% (21 original drugs). Of the 1,799 approved small molecule drugs listed in DrugBank, only 296 (16%) are anti-infectives and 202 (11%) are chemotherapeutics. This indicates a higher prevalence of prodrugs in the categories of anti-infectives and chemotherapeutics compared to the overall drug library. From 2018 to 2022, several prodrugs were approved for tropical infectious diseases, including tafenoquine and artesunate for malaria treatment, and propofol for tuberculosis treatment.Chemical Diversity of Prodrugs
A comprehensive analysis of prodrugs offers a thorough understanding of their distinctions from their APIs, thus defining the design space for prodrugs. The biotransformation mechanisms of prodrugs can be broadly categorized into three types: Prodrugs with Additional Atoms: These prodrugs include extra atoms compared to the API and require cleavage of promoieties to release the API. An example is fospropofol (Figure 1b). Prodrugs as Substructures of APIs: This type of prodrug represents a substructure of the API and requires metabolic addition to produce the API. An example is ganciclovir. Prodrugs Involving Molecular Rearrangement and Substitution: These prodrugs generate the API through molecular rearrangement and substitution. An example is omeprazole (Figure 3f).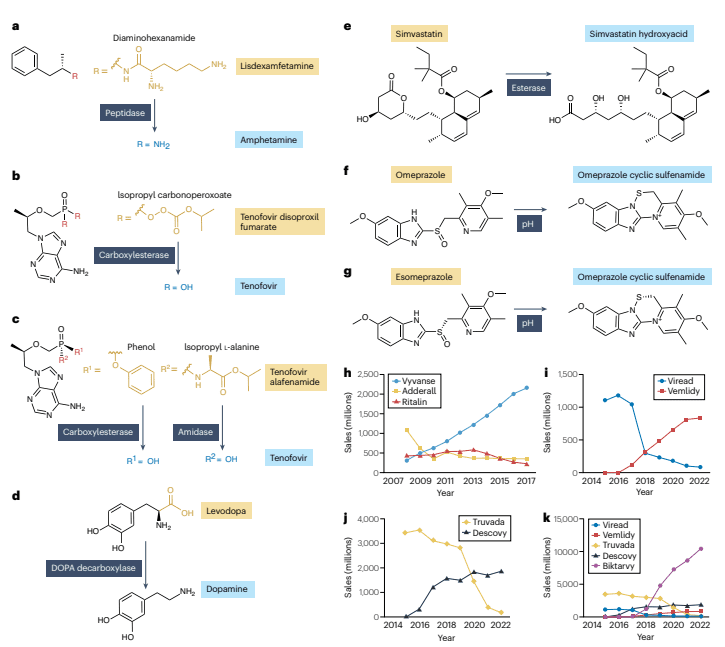
Figure 3: Examples of technical products that have a significant impact on the market
The chemical diversity of prodrugs is primarily reflected in the types and structures of the promoieties employed. Among the 178 FDA-approved prodrugs, 57% are larger derivatives achieved by attaching promoieties to the active pharmaceutical ingredient (API), which are then cleaved by endogenous mechanisms to release the API. These prodrugs utilize only 56 different promote ties. Most of these are aliphatic groups (73%), including simple alkyl chains and esterified alkyl chains, followed by aromatic rings (16%) and phosphate groups (11%). Additionally, specific functional groups are frequently used to optimize physicochemical properties, such as phosphate groups for improved solubility and aliphatic chains for enhanced permeability (Figure 1b, c). Overall, although the currently approved prodrugs exhibit relatively limited chemical diversity, they often employ simple promoieties in their design. These simple promoieties can serve as "workhorses" for future prodrug development.Biotransformation of Prodrugs to APIs
An analysis of 178 FDA-approved prodrugs revealed that 94% of the biotransformation processes are enzymatic reactions, with hydrolases being the most prevalent, particularly esterases and phosphatases. Oxidoreductases and kinases also significantly contribute to prodrug activation. Non-enzymatic transformations account for 6% and include pH-dependent and glutathione-involved reactions. Specific nitroreductases are utilized for targeted activation within the gastrointestinal tract. Half-life analysis of these prodrugs indicates that most are designed for rapid conversion to the active pharmaceutical ingredient (API). However, some prodrugs are formulated as sustained-release to extend the duration of the API's action. The release rate and half-life of prodrugs are influenced by the route of administration. Intravenous prodrugs generally exhibit a short half-life, while oral prodrugs often have a longer half-life, likely due to first-pass metabolism in the intestine or liver. Additionally, clearance and volume of distribution (Vdss) data show that prodrugs tend to have higher Vdss, which may be associated with their targeted properties.Physicochemical Properties of Prodrugs
The development of prodrugs aims to enhance the ADME (absorption, distribution, metabolism, and excretion) properties of the active pharmaceutical ingredient (API) by making precise adjustments to its physicochemical characteristics. In the study, 30 prodrugs designed to improve cell penetration exhibited a significant increase in lipophilicity compared to their corresponding APIs. Conversely, 13 prodrugs intended to enhance solubility demonstrated a decrease in lipophilicity. This indicates that even seemingly straightforward improvements require careful, case-by-case consideration. An analysis of 102 approved Class 1 prodrugs revealed that the molecular weight of the added promoieties ranged from 15 to 280 Da, with an average of 84 Da. The size of these promoieties was not directly related to the size of the API, but rather to their specific function in improving the drug's properties.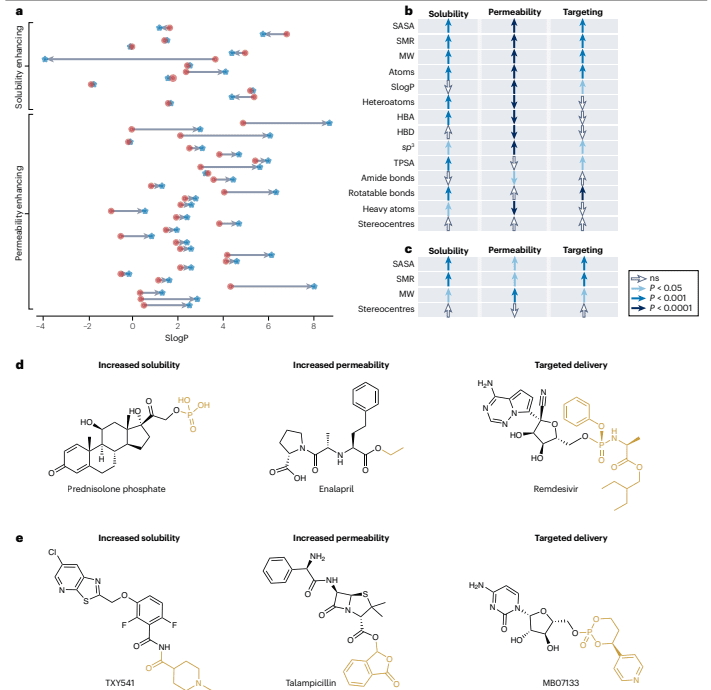
Figure 4: Physical and chemical properties of currently approved technical products
Currently approved prodrugs, despite generally featuring smaller and simpler chemical modifications, tend to have physicochemical properties similar to their APIs. However, they are often more complex and possess larger molecular weights compared to investigational prodrugs. This trend suggests a gradual shift in prodrug development from simple functionalization towards adopting more intricate chemical structures. The goal is to achieve more substantial pharmacokinetic and functional enhancements, such as targeted delivery, reduced toxicity, and sustained release, to meet specific design objectives.Conclusion
As drug design chemistry becomes more intricate, the use of prodrug strategies is expected to become more prevalent. Currently, most approved prodrugs focus on enhancing solubility or permeability. However, an increasing number of investigational prodrugs are being developed to tackle more complex ADME challenges. By rationally designing prodrugs to encompass a broader and more complex chemical space, future designer prodrugs could offer tailored pharmacokinetic profiles, paving the way for personalized medicine.References
- Fralish Z, Chen A, Khan S, Zhou P, Reker D. The landscape of small-molecule prodrugs. Nat Rev Drug Discov. 2024 May;23(5):365-380. doi: 10.1038/s41573-024-00914-7. Epub 2024 Apr 2. PMID: 38565913.
- Huang L, Yang J, Wang T, Gao J, Xu D. Engineering of small-molecule lipidic prodrugs as novel nanomedicines for enhanced drug delivery. J Nanobiotechnology. 2022 Jan 24;20(1):49. doi: 10.1186/s12951-022-01257-4. PMID: 35073914; PMCID: PMC8785568.
- Han HH, Wang HM, Jangili P, Li M, Wu L, Zang Y, Sedgwick AC, Li J, He XP, James TD, Kim JS. The design of small-molecule prodrugs and activatable phototherapeutics for cancer therapy. Chem Soc Rev. 2023 Feb 6;52(3):879-920. doi: 10.1039/d2cs00673a. PMID: 36637396.
- Li H, Zang W, Mi Z, Li J, Wang L, Xie D, Zhao L, Wang D. Tailoring carrier-free nanocombo of small-molecule prodrug for combinational cancer therapy. J Control Release. 2022 Dec;352:256-275. doi: 10.1016/j.jconrel.2022.10.022. Epub 2022 Oct 25. PMID: 36272660.