Tetracycline: A Versatile Antibiotic Facing Modern Challenges and Innovations
Abstract
Tetracycline is a broad-spectrum antibiotic known for its effectiveness against a wide range of bacterial infections, including both Gram-positive and Gram-negative pathogens. Discovered over six decades ago, Tetracycline has remained a cornerstone in the treatment of various infections due to its proven clinical safety, acceptable tolerability, and availability in both intravenous and oral formulations. However, the emergence of antibiotic resistance presents significant challenges, limiting its clinical utility. This blog post provides an in-depth look at the mechanism of action of Tetracycline, explaining how it interferes with bacterial protein synthesis. It also explores the various resistance mechanisms that have developed over time, including efflux pumps, ribosomal protection, and enzymatic inactivation. Recent advancements in Tetracycline research have led to the development of new derivatives with improved efficacy against resistant strains, offering hope for future applications. Our company is at the forefront of providing high-quality Tetracycline and related chemicals, including impurities, metabolites, isotope-labeled compounds, and building blocks, to support advanced pharmaceutical and biochemical research. With our comprehensive product range and commitment to quality, we aim to facilitate innovative solutions in the fight against antibiotic-resistant infections.
Introduction to Tetracycline
Tetracycline is a broad-spectrum antibiotic that has been a critical tool in the fight against bacterial infections since its discovery. It was first identified in the late 1940s from a soil bacterium, and its introduction revolutionized the treatment of various infections. Tetracycline and its derivatives are effective against a wide range of bacteria, including Gram-positive and Gram-negative organisms, making it a versatile and widely used antibiotic. Historically, Tetracycline was derived from the fermentation of Streptomyces aureofaciens, and it was marketed as Aureomycin. This discovery was followed by the identification of other tetracyclines, such as oxytetracycline and doxycycline, which have slightly different chemical structures but similar broad-spectrum activity. Over the years, these antibiotics have been used to treat numerous bacterial infections, including respiratory tract infections, urinary tract infections, skin infections, and sexually transmitted diseases. Tetracycline works by inhibiting bacterial protein synthesis. It binds to the 30S ribosomal subunit, blocking the attachment of aminoacyl-tRNA to the mRNA-ribosome complex, thereby preventing the addition of new amino acids to the growing peptide chain. This mechanism effectively halts bacterial growth, making Tetracycline bacteriostatic rather than bactericidal. Despite its efficacy, the widespread use of Tetracycline has led to the development of resistance. Bacteria have evolved various mechanisms to evade the effects of Tetracycline, including the production of efflux pumps that expel the antibiotic from the cell, ribosomal protection proteins that prevent Tetracycline from binding to the ribosome, and enzymatic inactivation. These resistance mechanisms have limited the clinical utility of Tetracycline, necessitating the development of new derivatives and alternative treatments. In addition to its medical uses, Tetracycline has found applications in veterinary medicine and agriculture. It is used to treat bacterial infections in animals and as a growth promoter in livestock, though its use in agriculture has been scrutinized due to concerns about antibiotic resistance. Our company is dedicated to providing high-quality Tetracycline and related compounds, including impurities, metabolites, isotope-labeled compounds, and building blocks, to support ongoing research and development in the pharmaceutical industry.Mechanism of Action and Applications
Tetracycline’s primary mechanism of action involves inhibiting bacterial protein synthesis, a process essential for bacterial growth and replication. This antibiotic binds to the 30S subunit of the bacterial ribosome, obstructing the attachment of aminoacyl-tRNA to the mRNA-ribosome complex. By preventing the addition of new amino acids to the growing peptide chain, Tetracycline effectively halts bacterial protein synthesis, leading to a bacteriostatic effect. This means it inhibits the growth and multiplication of bacteria without necessarily killing them, allowing the host's immune system to eliminate the infection.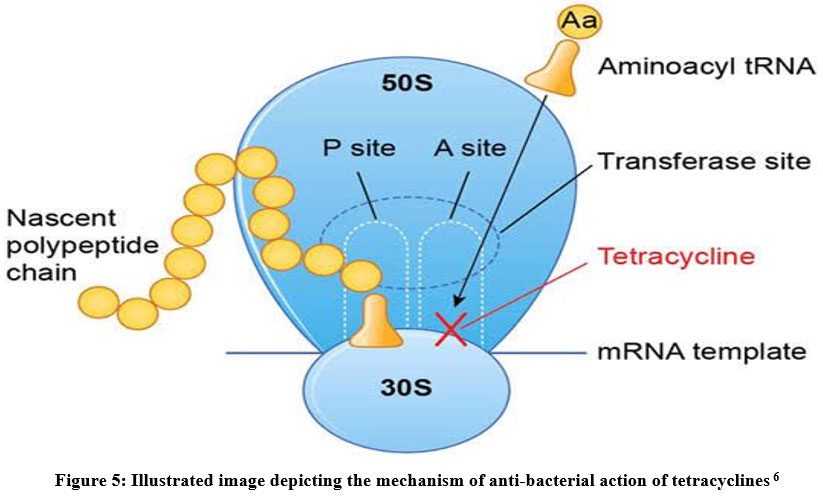
Resistance Mechanisms and Challenges
The widespread use of Tetracycline over the past several decades has led to the development of various bacterial resistance mechanisms, posing significant challenges to its clinical efficacy. Resistance to Tetracycline can occur through several mechanisms, including efflux pumps, ribosomal protection proteins, and enzymatic inactivation. One of the primary resistance mechanisms is the use of efflux pumps. These proteins, embedded in the bacterial cell membrane, actively expel Tetracycline from the cell, reducing its intracellular concentration and thereby diminishing its effectiveness. Efflux pumps such as Tet(A) and Tet(K) are commonly found in Gram-negative and Gram-positive bacteria, respectively. These pumps are encoded by genes that can be transferred between bacteria, facilitating the spread of resistance.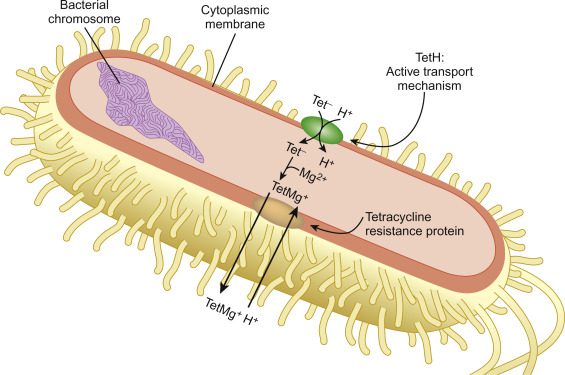
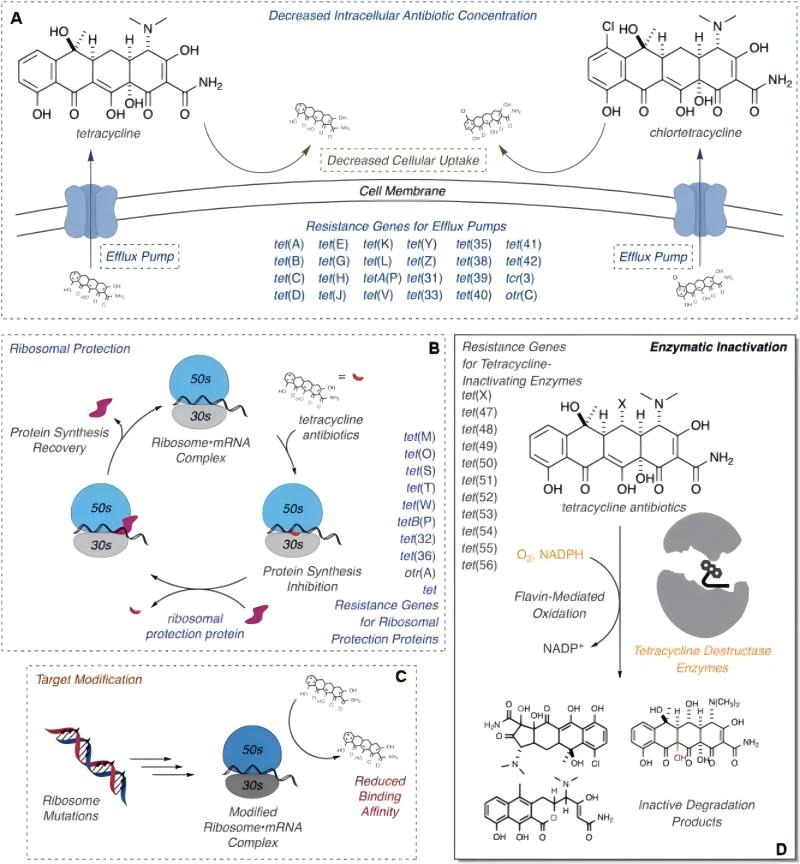
Advances in Tetracycline Research
Recent advancements in Tetracycline research have focused on overcoming resistance mechanisms and improving the antibiotic's efficacy. Scientists are exploring various chemical modifications and developing new derivatives to address the challenges posed by bacterial resistance. These efforts aim to enhance the drug's potency, spectrum of activity, and pharmacokinetic properties. One of the most significant advancements is the development of glycylcyclines, a new class of antibiotics derived from Tetracycline. Tigecycline, the first glycylcycline approved for clinical use, exhibits potent activity against a wide range of multidrug-resistant (MDR) pathogens, including those resistant to traditional Tetracyclines. Tigecycline is effective against Gram-positive and Gram-negative bacteria, anaerobes, and atypical pathogens, making it a valuable option for treating complicated infections.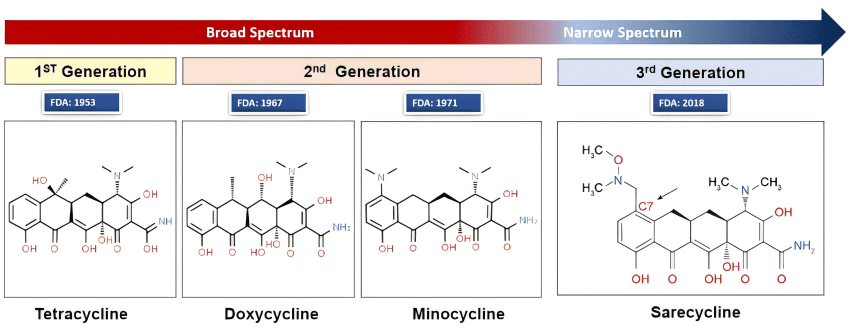