Things about Small Molecule Covalent Inhibitors
Abstract
This blog post explores the groundbreaking impact of small-molecule covalent inhibitors on drug discovery, highlighting their mechanisms, advantages, and recent developments. Covalent inhibitors form permanent bonds with target proteins, offering high specificity and prolonged therapeutic effects. The article delves into the categories of covalent inhibitors, including conventional inhibitors, targeted covalent inhibitors (TCIs), and expanded TCIs, and their applications in treating cancer, infectious diseases, and other conditions. Recent FDA approvals and emerging drug candidates underscore the growing importance of covalent inhibitors in modern medicine. The post also addresses the challenges of selectivity and outlines future directions for expanding the therapeutic scope of these powerful agents.
Introduction to Covalent Inhibitors
What are Covalent Inhibitors?
Covalent inhibitors are a class of drugs that form a permanent, covalent bond with their target proteins. This mechanism of action differentiates them from non-covalent inhibitors, which bind temporarily. Historically, covalent inhibitors were discovered serendipitously, with aspirin and penicillin being prime examples. These early discoveries set the stage for the significant therapeutic potential of covalent drugs, which have since become a focus of rational drug design due to advances in crystallography, computational chemistry, and bioinformatics.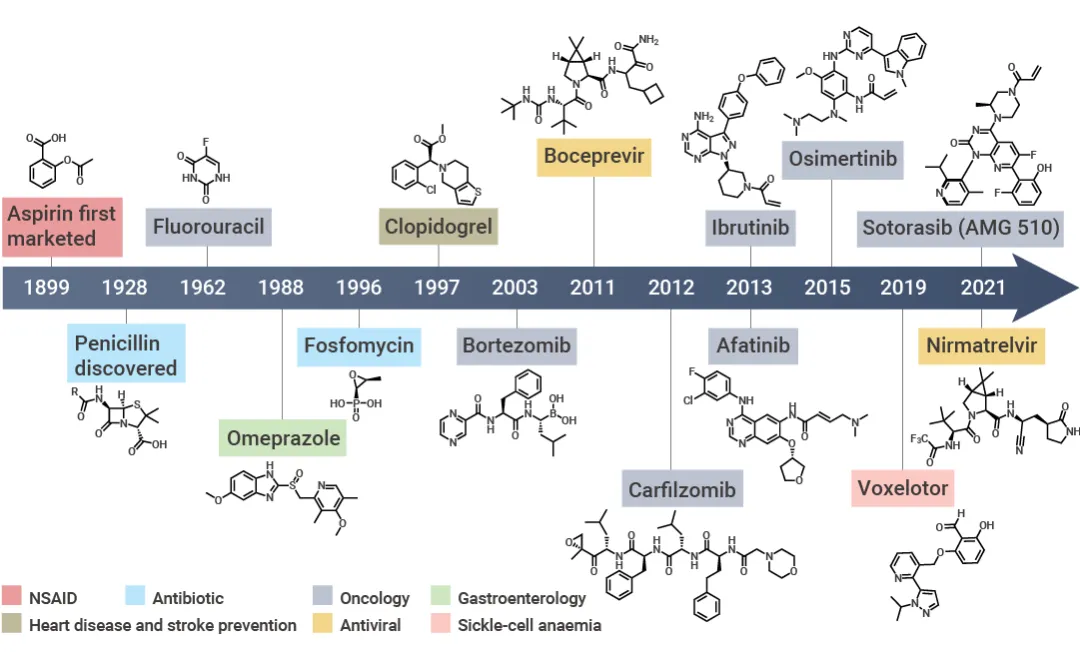
Figure 1. Development process of covalent drugs
Advantages of Covalent Inhibitors
One of the primary advantages of covalent inhibitors is their ability to achieve high binding affinity through the formation of covalent bonds. This strong binding can result in a prolonged duration of action, decoupling pharmacodynamics from pharmacokinetics and allowing the drug to maintain its therapeutic effect for extended periods. Additionally, modern covalent inhibitors have been designed with high target selectivity, which helps mitigate concerns regarding off-target effects and toxicity. This selectivity is crucial in reducing side effects and increasing the efficacy of treatments. Covalent inhibitors are particularly valuable in targeting proteins that were previously considered "undruggable." By forming a stable covalent bond with a specific amino acid residue on the target protein, these inhibitors can effectively modulate the function of proteins that play critical roles in disease processes. This capability opens new therapeutic avenues, particularly in areas such as oncology and infectious diseases.Categories of Covalent Inhibitors
Conventional Covalent Inhibitors
Conventional covalent inhibitors are among the oldest and most established types of covalent drugs. These inhibitors target the catalytic residues of enzymes, such as serine, threonine, and cysteine, which are critical for the enzyme’s function. Aspirin and penicillin are classic examples of this category. Aspirin acetylates a serine residue in cyclooxygenase (COX) enzymes, thereby inhibiting the synthesis of prostaglandins, which are involved in inflammation and pain. Penicillin, on the other hand, targets bacterial transpeptidase, an enzyme vital for bacterial cell wall synthesis, by forming a covalent bond with a serine residue in its active site.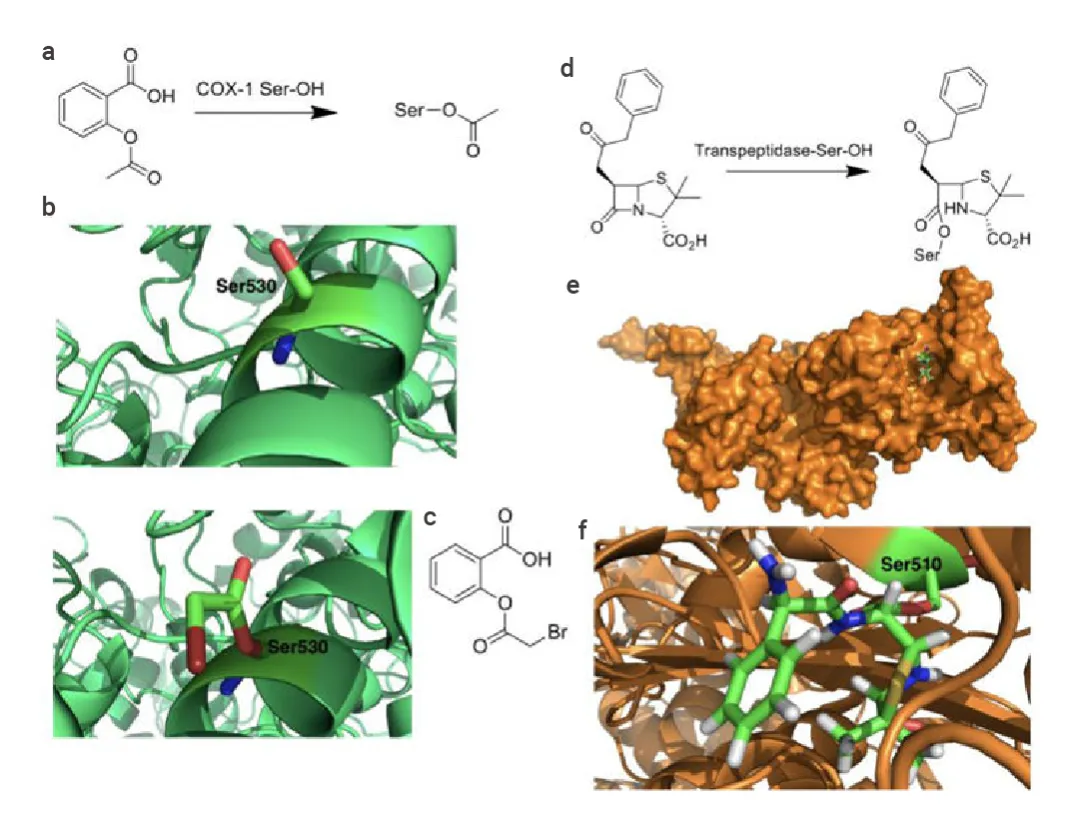
Figure 2. Diagram of the covalent mechanism of action of (a-c) aspirin and (d-e) penicillin.
Conventional covalent inhibitors are highly effective due to their strong and often irreversible binding to target enzymes, which can lead to prolonged therapeutic effects. However, this strong binding can also pose challenges, such as off-target effects and potential toxicity. The high conservation of catalytic residues across protein families can make it difficult to achieve specificity, increasing the risk of off-target interactions.Targeted Covalent Inhibitors (TCIs)
Targeted covalent inhibitors (TCIs) are designed to overcome the specificity challenges associated with conventional covalent inhibitors. Introduced by Singh et al. in 2011, TCIs achieve selectivity by targeting poorly conserved and non-catalytic residues, which are less likely to be shared among similar proteins. This approach allows TCIs to bind selectively to their intended targets, reducing the likelihood of off-target effects. Ibrutinib is a prime example of a TCI. It selectively inhibits Bruton’s tyrosine kinase (BTK) by covalently modifying a cysteine residue that is not conserved in other kinases. This selective targeting makes ibrutinib an effective treatment for certain types of lymphoma and leukemia, with fewer side effects compared to non-selective inhibitors.Expanded TCIs
Expanded TCIs represent the latest advancement in covalent inhibitor design. These inhibitors target novel therapeutic areas by modifying residues other than the commonly targeted cysteine, such as lysine, which is more prevalent in the human proteome. Additionally, expanded TCIs explore targeting allosteric sites and protein-protein interactions, broadening the range of potential therapeutic targets. By diversifying the types of residues and sites they target, expanded TCIs can address a wider array of diseases. For example, targeting allosteric sites allows for the modulation of protein function without directly interfering with the active site, which can lead to highly selective and effective therapies.Recent Developments in Covalent Inhibitors
FDA-Approved Covalent Inhibitors
In recent years, several covalent inhibitors have gained FDA approval, underscoring their therapeutic potential and clinical significance. These approvals highlight the diverse applications of covalent inhibitors in treating various diseases, particularly cancer and infectious diseases. For instance, sotorasib, a covalent inhibitor targeting the KRASG12C mutation, has been approved for the treatment of non-small cell lung cancer (NSCLC). This approval marks a significant milestone as KRAS mutations were previously considered "undruggable." Other notable FDA-approved covalent inhibitors include mobocertinib and lapatinib, both of which target mutations in the epidermal growth factor receptor (EGFR) in NSCLC. These drugs specifically inhibit the EGFR exon 20 insertion mutations, which are resistant to earlier generations of EGFR inhibitors. Their approval provides new treatment options for patients with these specific genetic alterations, offering hope for improved outcomes.Emerging Drug Candidates
Beyond the currently approved drugs, there are several promising covalent inhibitors in various stages of clinical trials. These emerging drug candidates are designed to address unmet medical needs and expand the therapeutic applications of covalent inhibitors. For example, adagrasib is another KRASG12C inhibitor showing promise in clinical trials for treating NSCLC. Its development is being closely watched as it may offer an alternative or complementary option to sotorasib. Similarly, research is ongoing to develop covalent inhibitors targeting other challenging mutations and therapeutic areas. For instance, covalent inhibitors are being investigated for their potential to treat multi-drug resistant (MDR) bacterial infections. ETX0462, a novel antibiotic targeting multiple penicillin-binding proteins and beta-lactamases, is one such candidate showing promising preclinical results.Mechanisms of Action
How Covalent Inhibitors Work
Covalent inhibitors function by forming a stable, covalent bond with a specific amino acid residue in their target proteins. This bond is typically formed between the inhibitor and a nucleophilic residue such as cysteine, serine, or lysine. The covalent attachment results in irreversible inhibition of the target protein, effectively blocking its activity. This mechanism allows covalent inhibitors to achieve high specificity and potency, as the formation of a covalent bond significantly increases the binding affinity between the drug and its target. The process begins with the inhibitor approaching the active site of the target protein. The covalent bond formation involves a nucleophilic attack by the amino acid residue on an electrophilic group present in the inhibitor. This reaction is often facilitated by the unique chemical environment of the active site, which stabilizes the transition state and allows for the formation of a stable covalent complex. The result is a long-lasting inhibitory effect, as the covalent bond is difficult to break, ensuring sustained inhibition of the target protein.Targeting Non-Catalytic Residues
One innovative strategy in covalent inhibitor design is targeting non-catalytic residues, which are less conserved across protein families compared to catalytic residues. This approach, known as targeted covalent inhibition, enhances the selectivity of the inhibitors. By focusing on unique residues not involved in the catalytic activity, researchers can design inhibitors that selectively bind to the desired target protein without affecting similar proteins. This strategy reduces the risk of off-target effects and associated toxicities. Ibrutinib is a prime example of a covalent inhibitor that targets a non-catalytic residue. It inhibits Bruton’s tyrosine kinase (BTK) by covalently modifying a cysteine residue (Cys481) that is not conserved in other kinases. This selectivity makes ibrutinib an effective treatment for certain lymphomas and leukemias with fewer side effects compared to non-selective kinase inhibitors.Therapeutic Applications
Anticancer Agents
Covalent inhibitors have shown significant promise in oncology, particularly in targeting cancer-driving mutations that were previously considered "undruggable." For instance, sotorasib, a covalent inhibitor targeting the KRASG12C mutation, has revolutionized the treatment landscape for non-small cell lung cancer (NSCLC). By covalently binding to the mutated cysteine residue, sotorasib effectively inhibits the activity of the KRAS protein, which plays a crucial role in cancer cell proliferation and survival. Other examples include covalent inhibitors targeting epidermal growth factor receptor (EGFR) mutations in NSCLC. Drugs like osimertinib and mobocertinib specifically inhibit EGFR mutations that confer resistance to earlier treatments, providing new options for patients with these resistant cancers.Antibiotics and Antiviral Agents
Covalent inhibitors also play a critical role in the fight against infectious diseases. The antibiotic penicillin, which covalently inhibits bacterial transpeptidase, was one of the first covalent drugs and remains a cornerstone of antibacterial therapy. More recently, nirmatrelvir, a component of the COVID-19 treatment Paxlovid, has demonstrated the power of covalent inhibitors in antiviral therapy. Nirmatrelvir covalently binds to the main protease of SARS-CoV-2, preventing viral replication and significantly reducing disease severity. Covalent inhibitors targeting multi-drug resistant (MDR) bacterial infections are also in development. These novel antibiotics aim to overcome resistance mechanisms by covalently modifying essential bacterial enzymes, offering hope for effective treatments against MDR pathogens.Challenges and Future Directions
Overcoming Selectivity Challenges
One of the primary challenges in developing covalent inhibitors is ensuring target selectivity while minimizing off-target effects. Covalent inhibitors, by nature, form irreversible bonds with their targets, which can lead to unintended interactions with similar proteins. To address this issue, researchers are employing various strategies to enhance specificity. One approach involves designing inhibitors that target unique, poorly conserved residues within the target protein. By focusing on these distinct sites, the inhibitors can achieve high selectivity and reduce the likelihood of off-target interactions. Another strategy is to use advanced computational methods and structural biology to predict and validate the binding interactions between inhibitors and their targets. These techniques help in optimizing the design of covalent inhibitors to enhance their selectivity and efficacy. Additionally, incorporating time-dependent inhibition kinetics can further improve selectivity, as it allows for a more controlled and targeted approach to drug binding.Expanding the Therapeutic Scope
The potential applications of covalent inhibitors extend beyond oncology and infectious diseases. Researchers are exploring their use in treating autoimmune diseases, neurodegenerative disorders, and other conditions with high unmet medical needs. For instance, covalent inhibitors targeting specific enzymes involved in inflammatory pathways could offer new treatments for diseases like rheumatoid arthritis and multiple sclerosis. In neurodegenerative diseases, such as Alzheimer’s and Parkinson’s, covalent inhibitors can be designed to target and modify pathogenic proteins involved in disease progression. By developing inhibitors that can cross the blood-brain barrier and selectively bind to these proteins, researchers hope to create effective therapies that can halt or slow down neurodegeneration. Furthermore, the development of covalent inhibitors for non-enzymatic targets, such as protein-protein interactions (PPIs), represents a burgeoning area of research. PPIs play crucial roles in various biological processes and diseases, but they have been challenging to target with traditional small molecules. Covalent inhibitors offer a promising approach to modulating these interactions and developing novel therapies for a wide range of diseases.Conclusion
Covalent inhibitors represent a transformative approach to drug discovery, offering unique advantages in terms of binding affinity, selectivity, and duration of action. The recent advancements in the design and application of covalent inhibitors have opened new therapeutic avenues and provided effective treatments for previously challenging targets. However, achieving precise selectivity and minimizing off-target effects remain critical challenges that require ongoing research and innovation. The future of covalent inhibitors looks promising, with potential applications extending to various therapeutic areas beyond oncology and infectious diseases. By leveraging advances in computational chemistry, structural biology, and innovative design strategies, researchers are continually expanding the scope and efficacy of covalent inhibitors. These efforts hold the promise of developing more targeted, effective, and safe therapies that can address unmet medical needs and improve patient outcomes.References
- Lee, J., & Park, S. B. (2022). Extended applications of small-molecule covalent inhibitors toward novel therapeutic targets. Pharmaceuticals, 15(12), 1478.
- Singh, J., Petter, R. C., Baillie, T. A., & Whitty, A. (2011). The resurgence of covalent drugs. Nature Reviews Drug Discovery, 10(4), 307-317.
- Bauer, R. A. (2015). Covalent inhibitors in drug discovery: from accidental discoveries to avoided liabilities and designed therapies. Drug Discovery Today, 20(9), 1061-1073.
- Gehringer, M., & Laufer, S. A. (2019). Emerging and re-emerging warheads for targeted covalent inhibitors: applications in medicinal chemistry and chemical biology. Journal of Medicinal Chemistry, 62(12), 5673-5724.
- Gehringer, M., & Laufer, S. A. (2019). Emerging and re-emerging warheads for targeted covalent inhibitors: applications in medicinal chemistry and chemical biology. Journal of Medicinal Chemistry, 62(12), 5673-5724.
- Liu, Q., Sabnis, Y., Zhao, Z., Zhang, T., Buhrlage, S. J., Jones, L. H., & Gray, N. S. (2013). Developing irreversible inhibitors of the protein kinase cysteinome. Chemistry & Biology, 20(2), 146-159.